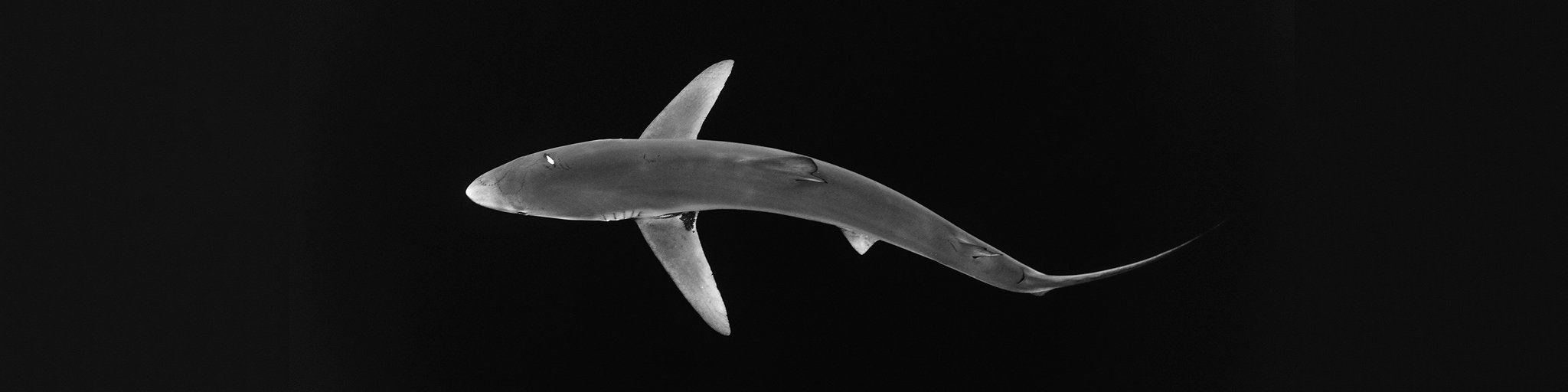
Thresher sharks: the biomechanics of tail-whipping
SHOW NOTES
We start by learning how Jamie got into the field. During her undergraduate in North Carolina, Jamie got the opportunity to participate in a ‘study away’ programme where she was based in Alaska for a semester [6.25]. This involved an internship that was somewhat outside the norm, called ‘Marine Animal Skeletal Articulation’. This immediately peaked Jamie’s interest, as previously she had worked with the Marine Mammal Stranding Programme in North Carolina, an organisation of people that are trained to respond to whale and dolphin strandings. She had always loved marine mammals and, in particular, their anatomy. The internship afforded Jamie the opportunity to learn more about this. In the sad event that a marine mammal does wash ashore, the skeleton is usually preserved for research purposes or for use in a museum. As an intern, Jamie would be helping to prepare the skeletons for these uses. During this time, Jamie’s supervisor wanted to give her ‘the whole Alaska experience’ and took her kayaking on the bay of a small town called Homer [8.47]. It wasn’t long until they heard the heart-stopping sound of a whale breathing, and a few minutes later a humpback surfaced right beside Jamie’s kayak! This is a moment she says that she will never forget, for the rest of her life.
The internship was not only memorable for that experience, but also because it set Jamie down the path that led her to where she is now. Although she had always been “obsessed” with whales [12.20], her first real introduction to skeletal anatomy was through her time in Alaska. Handling and processing so many whale vertebrae sparked a curiosity in Jamie – why were some vertebrae shaped a certain way? Why were some bigger than others? She soon wanted to understand how this translated into movement, and this led her to connect with her current PhD advisor Dr Marianne Porter at the Florida Atlantic Biomechanics Lab (AKA the FAB Lab!). And this is how sharks first came into the equation. Dr Porter’s work has a focus on sharks (she was a Save Our Seas Foundation Project Leader studying the movement ecology of blacktip sharks!) and she suggested that Jamie bring sharks into her research, alongside whales. At first, Jamie was more interested in whale vertebrae, but now believes shark vertebrae are “equally as cool” and “very fascinating” having spent some time studying them.
Jamie’s PhD is now focussed on the functional morphology of skeletal tissue – in a nutshell, how parts of the skeleton are shaped, and how that affects how the animal moves [17.01]. In particular, Jamie is interested in the smaller structures within a single vertebrae, called microstructures (which we’ll get into a little later on!). She looks at these for both sharks and whales, and tries to understand how these translate into movements like swimming, and in the case of thresher sharks, tail-whipping.
So, what are the differences between shark and whale vertebrae [19.47]? Whales have a vertebral column that is similar to our own, in that it is made of bone. Sharks, on the other hand, have a skeleton made of cartilage. This isn’t quite the same cartilage that humans have (the rubbery substance at the top of our ears, or the end of our noses). The cartilage in a shark’s skeleton is calcified, and scientists refer to it as ‘mineralised cartilage’. It’s very tough and durable, but much more flexible and lighter than bone. However, like us and like whales, a shark’s vertebral column is made up of many vertebrae lined up in sequential order, joined together. In sharks, they are connected by a joint; in marine mammals and humans, it’s a vertebral disc. Overall, shark vertebrae are cylindrical in shape and have an almost hourglass structure, with two cones on either side and an area in the middle called an ‘intermedialia’ (a very fun word to say out loud – have a go!). This is where the magic happens, so to speak – it’s where we see a lot of variation between species.
One example is a group of sharks called the lamniforms, or the ‘mackerel sharks’ [23.25]. This is a small but very diverse group, which include more unusual, filter-feeding species like the basking shark and megamouth, but also many of the very agile, fast-moving predators such as the white shark, the mako shark and the thresher. Jamie likes to think of these as the ‘Olympians’ of the shark world; the super athletes with their extreme physical capabilities. The intermedialia of these sharks is made up of plates of mineral, which Jamie describes as looking like the wheel of a bike, with the spokes radiating out from the centre. Basking sharks are the odd one out in this family [27.00]. Instead of having these ‘beautiful’ radiating plates of mineral, basking sharks had vertebrae so different that it made Jamie scratch her head! They are not mineralised at all, and have such a poor level of calcification in comparison to other sharks that Jamie couldn’t quite believe what she was looking at. Why? We aren’t quite sure yet – it remains a mystery, as many things are with the basking shark! Other sharks, like blue and lemon sharks, have a more wedge-like structure, so that they almost have a block of mineral. So, there is variation in the vertebrae between species. And sometimes, there is even variation between individuals – much like in humans.
One shark that Jamie has studied in depth is the thresher shark [33.35]. There are three species: the big-eye, the pelagic, and the common. Their distribution varies with the species, but in general they are found in tropical to temperate seas, usually offshore in open water. They are a very distinctive shark, with short, blunt snouts and large, round eyes which Jamie thinks makes them look perpetually anxious! But their most obvious identifying features are their fins, which are incredibly elongated in comparison to other species. This includes the pectoral fins, but also the tail. In many species of shark the top half of the tail is longer than the bottom (this is known as a ‘heterocercal tail’), but thresher sharks really take that to the extreme. The upper lobe of the tail can be as long as the shark itself, giving it a ‘scythe-like’ appearance. But aside from looking strange, the tail has a purpose: to stun fish. The thresher uses it like a whip, snapping it overhead so fast that it creates a shockwave capable of affecting multiple fish at once. Scientists have estimated that they can bend their tails 180 degrees in just one third of a second! The thresher will swim at a shoal of fish at high speed, before using those long pectoral fins to brake suddenly so that their backend lifts up (much like if you slam the front brakes on a fast-moving bicycle). They then use that momentum to move their elongated tail overhead, where it snaps like a whip.
Jamie’s research sought to understand how the thresher’s vertebral column can support such an extreme movement, but this only became possible after a specimen became available through NOAA [41.22]. All three species of thresher are classified as threatened with extinction by the IUCN Red List, and so opportunities to study them are hard to come by. The specimen that Jamie received was so precious that she used ‘non-destructive’ methodologies to ensure the samples could be re-used. This included micro-CT scanning, a type of X-Ray that generates a 3D image. Jamie describes this as a ‘digital dissection’, where you can use a computer to look inside the animal rather than having to physically cut it open.
And what Jamie was able to find is fascinating [45.38]. First, she looked at the micro-structures – think back to the bike-wheel we were discussing earlier, the intermedialia with its plates of mineral. Each one of those plates can sometimes branch, and if the branch splits, that is known as a ‘node’. Jamie counted how many nodes she found within each plate, and where they were within the vertebrae. She also looked at the macrostructure, or the size and shape of each vertebrae in different parts of the vertebral column. Jamie found that in the main body of the shark, the vertebrae were taller, wider and longer, with more nodes or branching (but less plates of mineral). In the back section, or the tail end, the opposite was true: vertebrae were smaller with less nodes, but more plates. Jamie and her team theorised that this is because bigger and longer vertebrae provide more stability, whereas more numerous, shorter vertebrae would give more flexibility. If we liken our thresher shark to a catapult, then the body of the shark would be the beam – a stiff, strong structure that can provide a stable base. Here, the high level of branching almost acts like the struts on a bridge, giving extra support to stabilise the shark. The tail is then the payload or ‘sling’ of a catapult, which needs to be flexible in order to bend so extremely.
Jamie was also able to look at different age groups and identify differences between juveniles and adults [50.22]. Juveniles had a slightly larger body than their tail, with less microstructures than adults. To understand why, we need to dive into some physics – more specifically, something called the ‘kinetic link principle’. You might think that having a longer, heavier tail might make tail-whipping more cumbersome for adults, but in actual fact the opposite is true. If we go back to our catapult analogy, we know that catapults with longer slings will strike quicker than a catapult with a short sling. If we translate that to threshers, if adults grow to have a tail longer than their bodies, it actually puts them at an advantage; they can strike quicker and more efficiently with the same amount of effort.
Cool, eh? And that’s just the start of Jamie’s research – she is studying a number of different species, including marine mammals! You can follow along with her work on X/twitter (@CornOnTheKnaub) or on LinkedIn (Jamie Knaub).
ABOUT OUR GUEST
Jamie is a doctoral candidate in the Integrative Biology Program within the Department of Biological Sciences at Florida Atlantic University. Her research interests include functional morphology, anatomy, and biomechanics of skeletal tissue in large swimming vertebrates. Jamie’s dissertation research is focused on the microstructures within shark and cetacean (whale, dolphin, and porpoise) vertebrae. Additionally, she is the research technician at the FAU High School Owls Imaging Lab where she oversees high school students, college students (both undergraduates and graduates), PIs, and external collaborators who use the laboratory and equipment.
You can read the fascinating thresher shark paper here and find out more about the lab where Jamie is based, the FAB Lab, run by Dr Marianne Porter, here.
